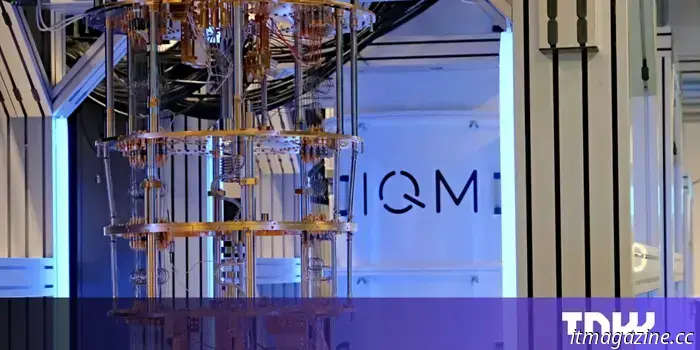
We entered IQM's quantum laboratory to observe a new realm of computing.
“The Future is Here,” states a striking neon sign at the entrance of IQM’s quantum data center in Munich. It's an ambitious assertion — one that the Finland-based startup is committed to realizing. To the right of the entrance sign is a sturdy, metallic blue door. My guide, physicist Frank Deppe, who is the head of quantum processing unit (QPU) technology at IQM, invites me inside.
Opened last year as part of IQM’s expansion into Europe, this facility houses six cutting-edge superconducting quantum computers, utilized for the company's own research and offered as a cloud-based service to scientists worldwide.
My first impression is the sound — a continuous, low purr interrupted by an unusual rhythmic pumping noise. Later, I would learn that this was the heartbeat of a quantum computer.
The centerpiece of the data center is the cryostats — the ornate, chandelier-like structures that have become iconic in the public’s perception of quantum computing.
Cryostats consist of a complex arrangement of gold-plated brass and copper wiring that directs microwave signals down to the QPU, or “chip,” located at the bottom of the chandelier. These microwave pulses enable scientists to manage and manipulate the qubits on the chip, facilitating the execution of algorithms for quantum calculations.
To function correctly, superconducting quantum computers must be cooled to nearly absolute zero (or -273.15 degrees Celsius), making these machines among the coldest places in the universe.
Qubits, the fundamental units of information in a quantum computer, are extremely sensitive to heat, vibration, stray particles, or electromagnetic signals. Even the slightest disruption can lead to errors or completely erase information, Frank explains, gesturing as though he can visualize the waves and particles around us.
At ultra-cold temperatures, superconducting materials eliminate all electrical resistance, allowing qubits to preserve their delicate quantum characteristics. However, ultra-cold alone is insufficient; qubits also require near-perfect isolation from surrounding particles in the air. This is why cryostats are placed within a thick metal vacuum chamber to shield the qubits from interference.
When active, the cryostat is secured inside a super-cooled vacuum chamber, making these machines some of the coldest places in the known universe.
Each machine is backed by substantial industrial hardware. The cryogenics system is one of the largest pieces of equipment in the lab, consisting of a network of compressors, tanks, pumps, and pipes tasked with delivering liquid helium to super-cool the cryostat. The helium compressor produces the characteristic rhythmic sound of a quantum computer, while the cryostat itself remains silent.
Additionally, servers are positioned next to each cryostat, providing the precise control and support infrastructure essential for the effective operation of delicate quantum systems. They also generate the specific microwave pulses necessary to stabilize the qubits.
Even future quantum computers will rely on classical computers to operate, Frank mentions.
I was struck by the vast amount of infrastructure required to power a quantum chip that is hardly larger than my fingernail. This technology is crucial — it safeguards the delicate qubits while still allowing for their manipulation.
“You need to isolate qubits from the environment — but still control them,” Frank states. “That’s the engineering paradox of quantum computing.”
Engaging with the complex subatomic world of quantum mechanics — with phenomena like superposition and entanglement — to accomplish practical computations is one of the greatest challenges in contemporary science that has puzzled researchers for decades. Yet now, following years of consistent advancement, we are closer than ever to potentially transformative applications, which could yield substantial benefits.
Advancing Towards Quantum Advantage
The future of quantum computers is anticipated to include capabilities far beyond the limits of today’s most powerful supercomputers — a concept referred to as “quantum advantage.” These machines could simulate intricate molecules for drug development, create new materials from an atomic perspective, and transform logistics and finance by solving extensive optimization challenges. They could also potentially compromise all internet encryption on what is known as Q-Day — presenting significant risks.
However, most experts concur that a system with 1 million qubits or more will be necessary to achieve such calculations — and we are still quite a distance from that milestone.
Presently, we are in what's termed the Noisy Intermediate-Scale Quantum (NISQ) era, characterized by small quantum computers capable of executing actual experiments but still too “noisy” and error-prone to achieve anything groundbreaking.
IQM’s quantum processors currently range from six to 50 qubits. Next year, the company plans to launch a larger system, Radiance, boasting 54 to 150 qubits, which it claims will “pave the way” to quantum advantage — when a quantum computer can resolve a challenge that no classical computer can. IQM aspires to develop a 1 million-qubit system by 2033.
Located in Helsinki, IQM has established a business that aids researchers in learning about and working with smaller
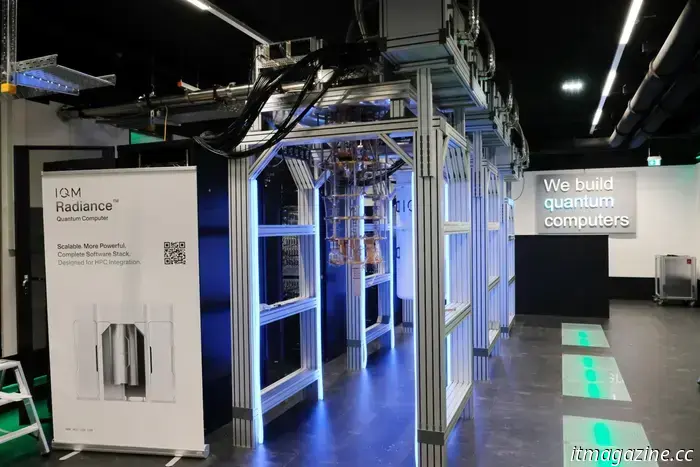
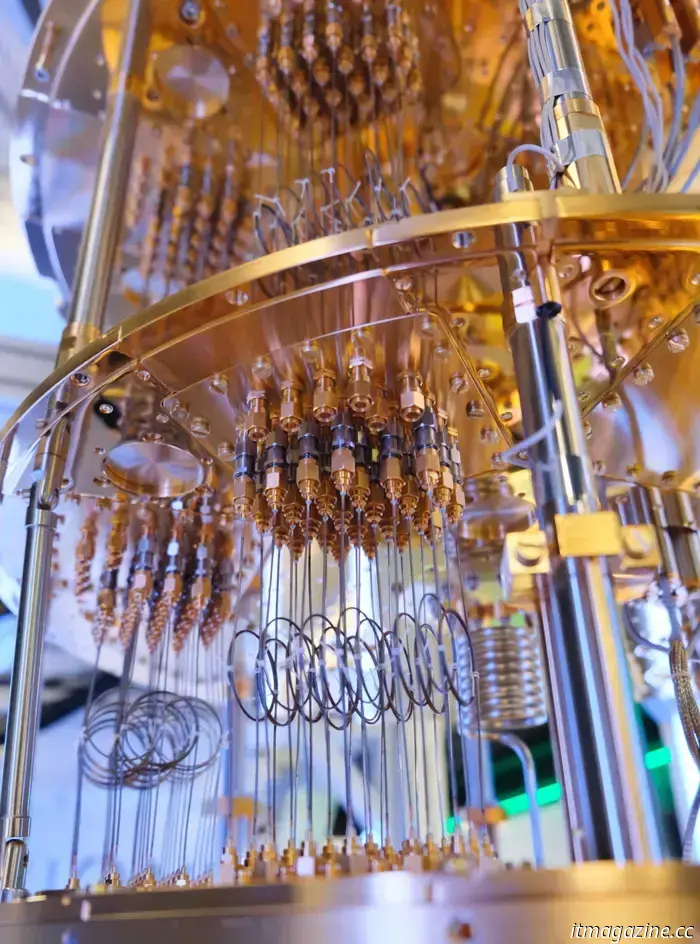
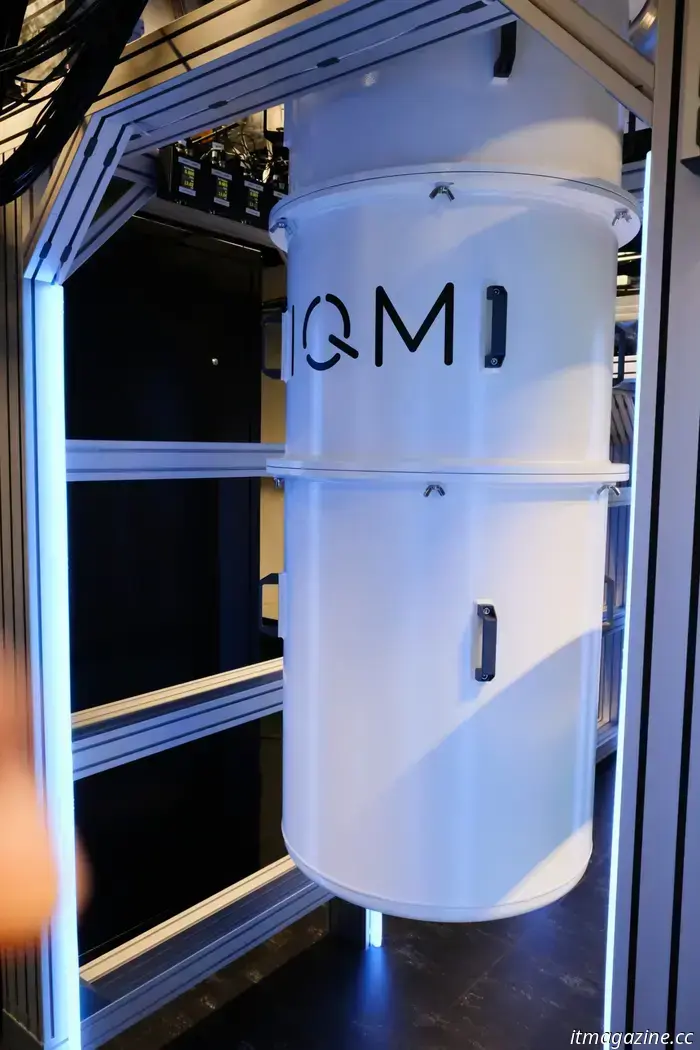
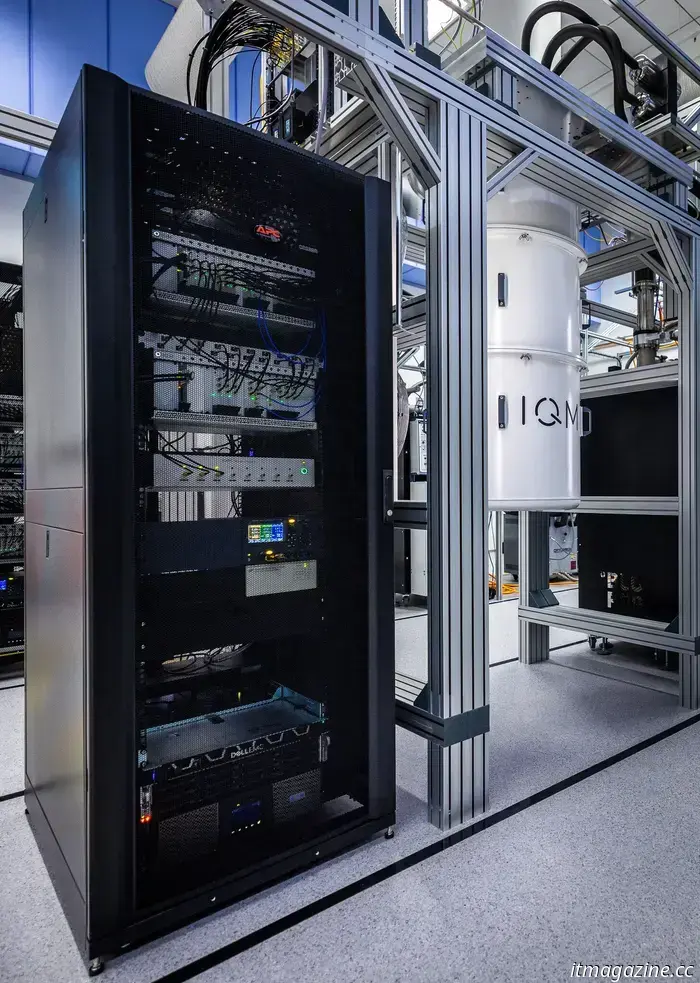
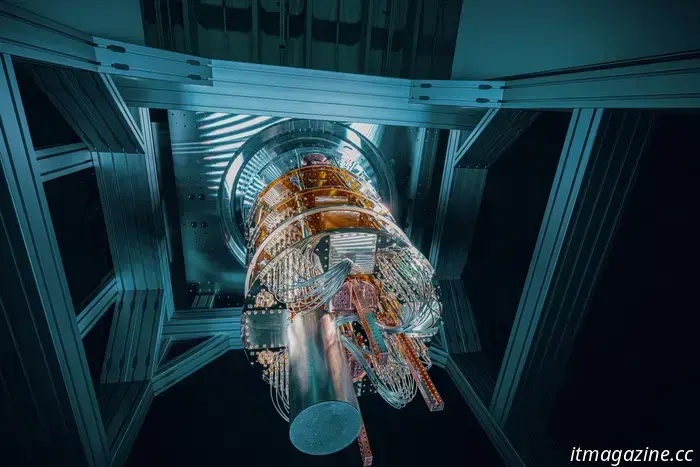
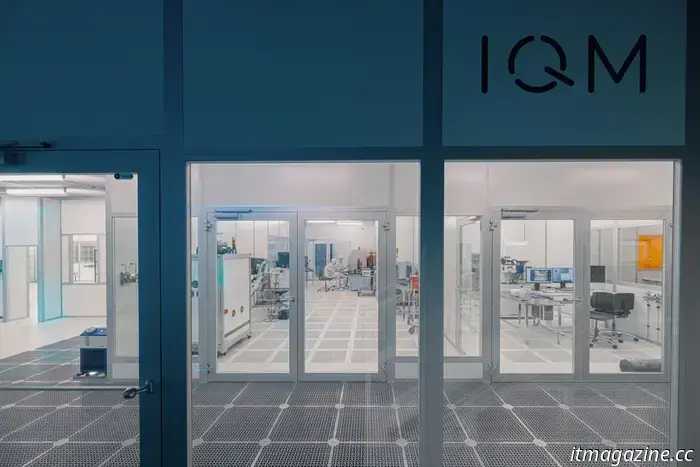
Other articles
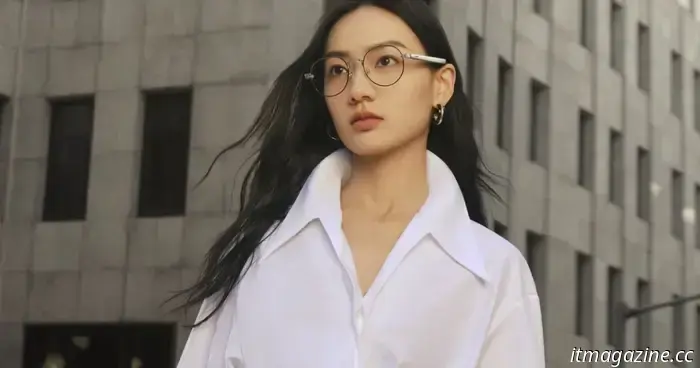
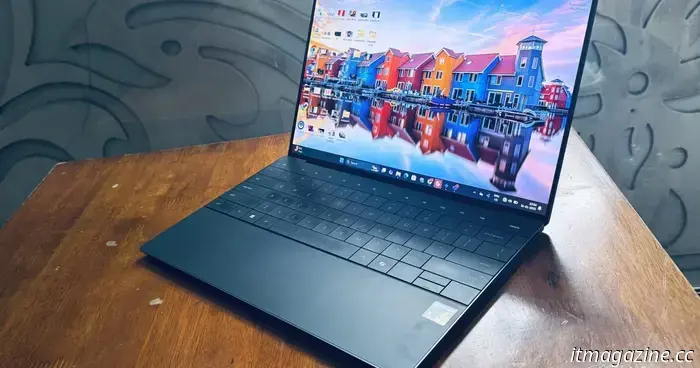
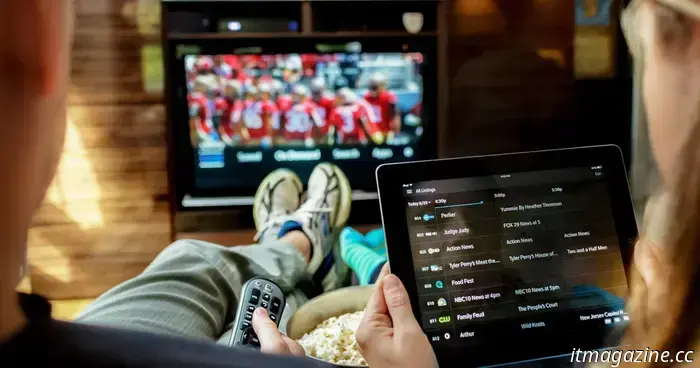
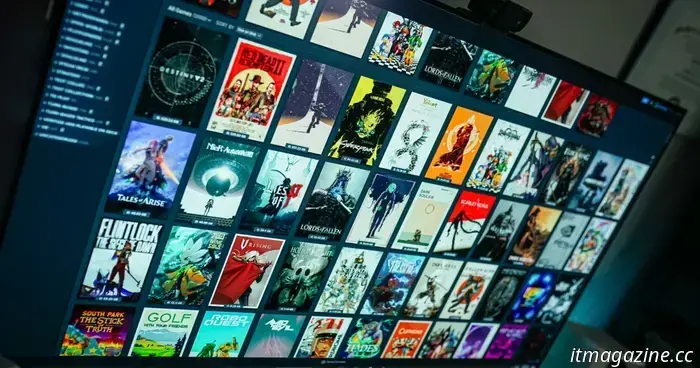
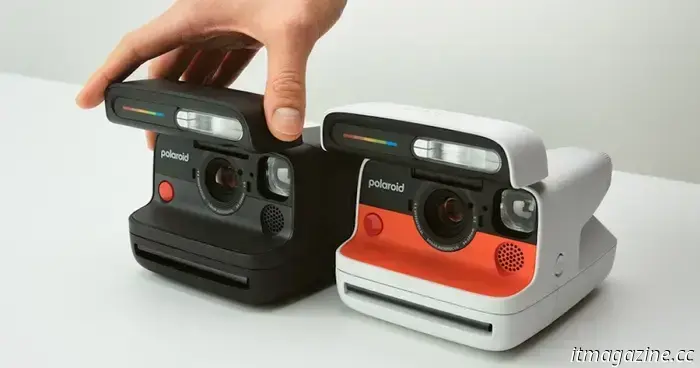
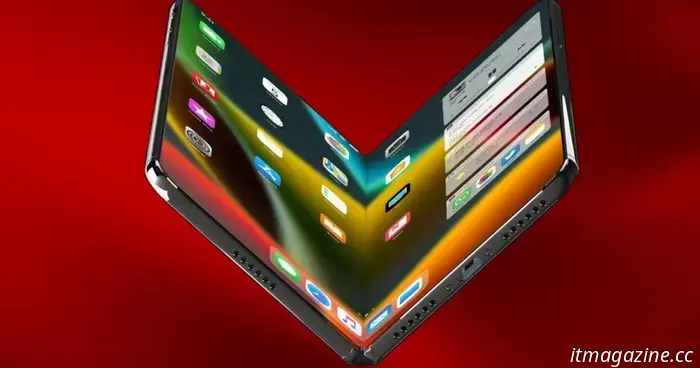
We entered IQM's quantum laboratory to observe a new realm of computing.
We visited IQM's quantum data centre in Munich to explore the hardware responsible for the astonishing phenomena of quantum mechanics.